As s&c coaches, how do we know we're going to make someone better?
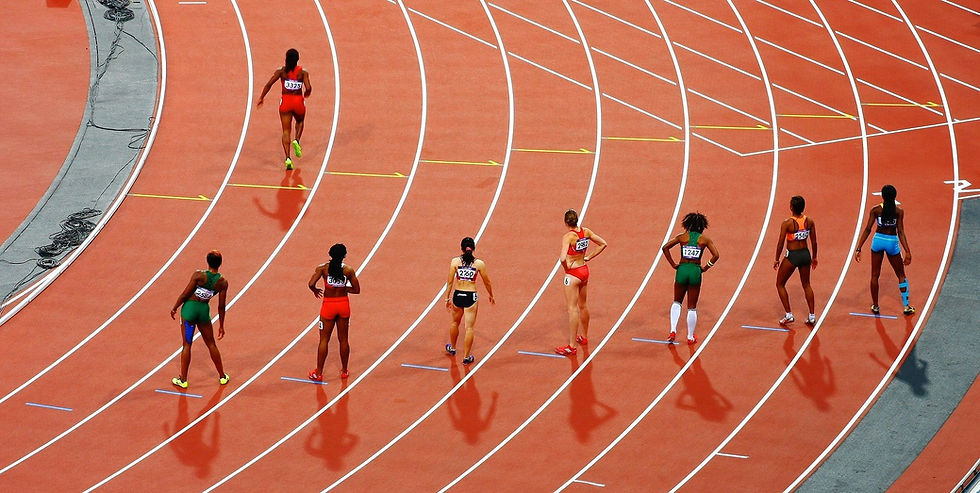
Answering this question usually starts with a needs analysis of the sport, the athlete, and how the two combine to find the things you need to work on. I think s&c coaches do the first two things very well but putting the recipe all together and making a 5 star dish at the end is often problematic.
Why? Two reasons, first understanding the complex interplay between all the variables and secondly, misunderstanding or not appreciating the role of strategy, tactics, styles, opponents, environment that have a large role to play in the execution of performance in many sports. Essentially, when we take a look at the sport and the athlete, how do we know we're seeing what we think we're seeing? And is it robust, consistent and stable?
In this example, even as something as simple as a 400m sprint, are we truly seeing genuine capacity limitations or just poor execution of race pace? Usually tactics are left to the skills coach, but this is where it is vital for the s&c coach to have some understanding of the sport.... or at least allow for an interdisciplinary team approach.
Below is some heavy reading, but I will run you through a sports analysis of the 400m sprint to highlight these issues.
The sport analysis
The 400m sprint is characterised by three key components; acceleration, maximal velocity, and the capacity to sustain maximal velocity over a given distance (Grgić et al., 2009; Thompson., 2017). The 400m sprint has been described as one of the most physically demanding track events in athletics due to the temporal and task demands. That is, aiming to complete the allotted distance in the least amount of time possible. The distance is typically completed in sub 45 and 53 seconds for elite male and female athletes respectively (Quercetani, 2005). This has led to the nomenclature in the literature referring to the 400m race as a 'long sprint', separating short sprint and middle distance events due to the complex interaction of biomechanical, physiological and race pacing strategies that underpin successful performance (Thompson, 2017). The biomechanical analysis of the 400m sprint is considered in relation to the key components. Desirable performance outcomes are dependent on a multitude of factors, most notably the athlete’s capacity to express force, flexibility, technique efficiency, anthropometrics and genetics. Spatiotemporal factors such as stride frequency and stride length are common kinematic descriptors of sprint performance in biomechanical literature (Goodwin, Tawiah-Dodoo, Waghorn, & Wild, 2018). Consequently, these kinematic components are often scrutinised when trying to enhance performance. A mathematical relationship exists between stride length andstride frequency. Theoretically improvements in both should amount to an overall increase in velocity however the relationship isn’t causal (Hunter et al., 2004; Monte et al., 2016). That is, stride lengths are a consequential outcome of increased velocity rather than a desired coachable strategy to increase velocity (Mann, 1984; Toyoshima et al., 2016). Furthermore, stride length can be a result based on anatomical factors such as leg length (Goodwin et al., 2018). Ultimately, research suggests the limits to maximum velocity are imposed from ground contact. This makes intuitive sense, as when the athlete is in flight, they cannot increase their velocity any further (Weyand, Sandell, Prime, & Bundle, 2010). The primary objective then for the athlete in acceleration is to maximise horizontal impulse in the shortest possible time (Goodwin et al., 2018). In short distance sprints acceleration has a more impactful role on the performance outcome (Mann & Murphy, 2018). However, it is prudent to consider that maximal velocity is a consequence of acceleration. That is, maximal velocity can be defined as the speed at which the athlete can nolonger accelerate (Goodwin, 2011). Therefore, quality acceleration enables the athlete to reach a higher maximal velocity which in turn is paramount to 400m success (Mann & Murphy, 2018). Force reigns supreme when considering the mechanical definition of acceleration; the greater net impulse the greater change in velocity (Cleather, 2018). However intuitively the expression of force is constraint by the task demands. Therefore, maximising the time spent on the ground and expressing force rapidly is paramount for the athlete (Weyand et al., 2000; Weyand et al., 2010). Optimisation of the contractile and elastic properties of the musculoskeletal system is therefore critical for performance. Absolute maximal strength levels in back squat type variants and slow stretch shortening cycle activities such as a countermovement jump are strongly correlated with acceleration performance (Čoh et al., 2013; Cronin et al; 2007; Mcbride et al., 2009; Sleivert et al., 2004; Styles et al., 2016; Wisloff, 2004). Whilst joint moments and sequencing differ during these movements, leg extension force capability is the common denominator (Goodwin et al., 2018). It is therefore commonplace to consider the musculature of the hip extensors, knee extensors and ankle plantar flexors profoundly dominant in the stance phase of acceleration (Morin et al., 2015a, 2015b). There are three ways inwhich impulse can be improved, an increase in total amount of maximal force, rate of force development and the duration of which force is applied. Research from Slawinski et al. (2010) suggested there were no significant differences between elite and sub-elite sprinters in overall duration of ground contact in acceleration, however the rate at which force was applied was found to have a significant difference between elite and sub elite sprinters. Therefore, the rate of force development (RFD) is a fundamental characteristic of acceleration performance (Cross et al., 2016; Slawinski et al., 2015). The kinematics of the acceleration phase are also considered. Optimising technique enables for efficient transfer for force, reducing unnecessary energy expenditure and therefore increases acceleration performance (Goodwin, 2011). This has been demonstrated with elite sprinters orientating their body with an increased horizontal vector in comparison to their sub elite counterparts. Therefore, the ability to direct force optimally was considered to be more impactful than overall total force applied (Morin, Edouard, & Samozino, 2011). Additionally, faster sprinters are able to sustain a greater horizontal vector for longer durations (Kugler et al.,2010; Morin et al., 2015). However, increasing the relative amount of horizontal force can only be achieved if the athlete is sufficiently strong, highlighting the interaction between skill and capacity (Goodwin et al., 2018). Whilst technique can be individual some common trends are seen in elite performers. Stride frequency during acceleration is considered vital as this minimises flight time and maximises the chance to accrue horizontal impulse (Mann & Murphy, 2018). Centre of mass to touchdown distance is often an indicator over the first initial steps to identify whether the athlete is achieving negative touchdown distance; ground contact in the first 2 steps should ideally be behind the centre of mass in order to propel the athlete forward as demonstrated by elite sprinters (Mero, Komi, & Gregor, 1992). Furthermore, Sufficient ankle dorsiflexion minimising yield on ground contact is also observed (Goodwin, 2011). As the athlete transitions from acceleration to maximal velocity, they must contend with further diminishing contact times. Maximal velocity consists of overcoming gravity and managing breaking force by producing as much vertical impulse as possible to maintain forward motion (Goodwin et al., 2018). Similarly, as with acceleration due to the task demands performance is largely dictated by the ability to produce substantial ground reaction forces relative to body mass in the least possible time. Ground reaction forces can reach up to five times bodyweight during stance, highlighting the physical demands on the musculoskeletal system (Udofa, Ryan, Clark, & Weyand, 2017). Due to the decreased time to produce force, the elastic properties of the musculoskeletal system contribute more to maximal velocity sprinting than acceleration, describing the limb in stance to be comparable to a stiff spring (Goodwin, 2011; Kuitunen et al., 2002). This is facilitated by the co-contraction of the calf and hamstring before touchdown in order to keep the knee in place allowing the hip to do work (Goodwin, 2011; Kyröläinen et al., 2005). This enables the athlete to resist yielding on ground contact promoting faster contact time. Therefore, it is proposed elite sprinters have a greater ability to generate higher eccentric forces, being able to decelerate and utilise greater elastic energy than sub elite counterparts. Research from Clark and Weyand (2014) demonstrate this characteristic in time force waveforms (Figure 1). A reactive strength index (RSI) profile (Table 1) is often administered within the gym environment to quantify fast stretch shortening cycle capability and therefore help assess potential sprint performance. RSI is derived from unilateral or bilateral jump assessments which primarily focus on the performance of the achilles tendon complex (Čoh & Mackala, 2013).
Figure 1. Force Time Waveform on Ground Contact

Note: Increased rate of force development demonstrated by elite sprinters in the first half of ground contact. The sharp inclination of the curve demonstrated by elite sprinters highlights the importance of the ability toproduce force rapidly. Graph adapted from Clark and Weyand (2014).
Table1. RSI Normative Data for Elite Sprinters
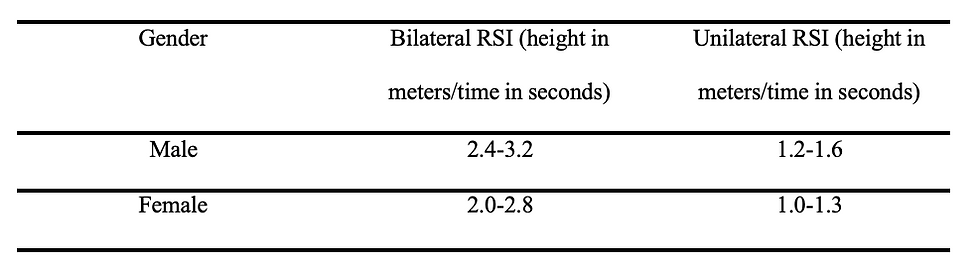
Note: Reprinted from Goodwin et al., (2018).
Similarly, movement kinematics are considered during maximal velocity sprinting. Whilst individual differences occur, foot placement on touchdown is recommended just in front of the centre of mass. This to avoid unwanted breaking forces, minimise potential hamstring injury and reduce contact length (Goodwin, 2011; Mann et al., 2016). Furthermore, as described in the kinetic characteristics, pre-tensing the lower limb before contact enables the foot to strike and utilise elastic properties. It is considered 10˚ of plantarflexion during mid stance is recommended to avoid the heel striking the track causing increased contact time and breaking forces (Goodwin etal., 2018). Moreover, the swing leg is also considered; the rapid reflexive flexor extensor pattern is vital for force production at maximal velocity. The hip flexor at toe off forcefully pulls the knee through during swing inpreparation for ground contact (Bosch & Cook, 2015). The differences between acceleration and maximal velocity are summarised in Table 2.
Table 2. Summary of Differences Between Acceleration and Maximal Velocity Phases of
Sprinting.
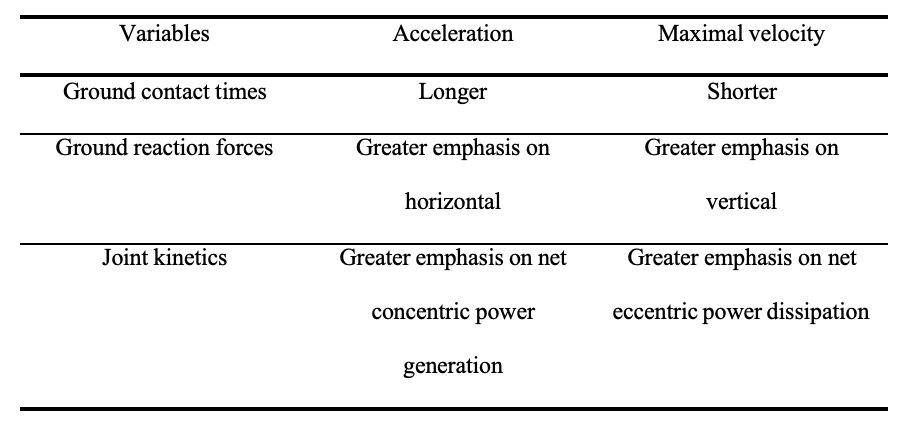
Note: Reprinted from Wild, Bezodis, and Blagrove (2011).
Once the athlete has accelerated and can no longer accelerate, the ability to maintain maximal velocity for the remainder of the duration is the biggest challenge. Due to the energetic limitations of the ATP- PCr system the 400m sprint cannot be performed at maximal effort with no consideration for fatigue (Mann & Murphy, 2018). Ultimately, the important interaction between maximal force production, efficient mechanics and energetics are highlighted during this crucial phase. Race pace tactics are therefore clearly a significant consideration for the athlete and coach within the needs analysis. In research presented by Hanon and Gajer (2009) world class sprinters revealed a more aggressive pacing strategy than their sub elite peers, with the first 200m of the race performed at 97% of their 200m personal best (PB). Furthermore, two other commonalities were observed; a progressive decrease of velocity towards 300m and a further dramatic decrease in the last 100m. Additionally, elite sprinters had a greater decrement in velocity over the last 100m than sub elite sprinters. It is therefore supported that the ability to reach higher velocities over shorter distances is crucial for 400m success. That is, the faster the sprinter can travel in the early stages of the race will increase the margin for error in the latter part of the race. However, anecdotally it is uncommon for there to be an elite performer competing in both 100m and 400m sprint events. It can be summarised; it is a requirement for the 400m sprinter to be sufficiently fast to optimise their chances for success (Grgić et al., 2009; Hanon et al., 2009; Mann et al, 2016). Consequently, understanding the implications when trying to adopt this strategy is paramount for coaches. That is, pushing a certain percentage of an athletes 200m PB may not be currently optimal for that individual based on their strengths and weaknesses. Therefore, the strategy that achieves the fastest time should be considered the best strategy regardless of how it is achieved. It is then evident that understanding the physiological requirements of the 400m sprint will assist with optimal coaching and racing strategies. The physiological requirements are dictated by the temporal demands of the event and the nature in which it is performed. Contribution from the aerobic and anaerobic energy systems have been studied extensively in the literature (Table 3).
Table 3. Energy System Contribution to the 400m Sprint.
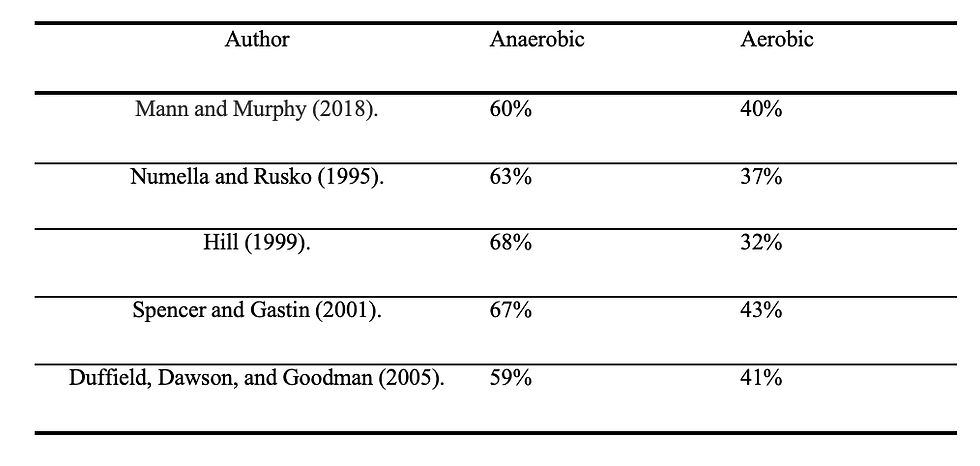
Note: The anaerobic systems consisting of the ATP-PCr and gylycotic pathways demonstrate a greaterpercentage contribution to 400m performance.
Furthermore, it was noted a crossover point between where anaerobic dominance starts to decline, this occurred at roughly 30-35 seconds into the event (Duffield, Dawson, & Goodman, 2005). This results in vast differences in kinetics and kinematics observed over the duration of the sprint. Comparing contact time and stride frequencybetween the first 100m and the last 100m demonstrates the impact of fatigue on elite performers (Figure 2).
Figure 2. Fatigue Impacting Stride Frequency and Ground Contact Time.
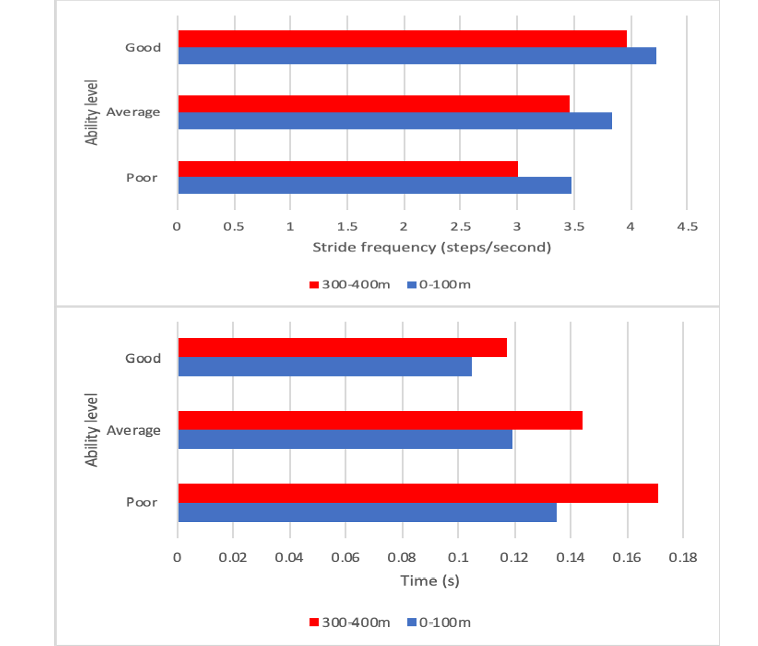
Note: Stride frequency deteriorates whilst ground contact time increases amongst all skill levels, demonstrating the impact of fatigue on biomechanical performance measures. Adapted from Mann and Murphy (2018).
The metabolic requirements for the athlete are then multifactorial, the rapid uptake and utilisation of ATP via anaerobic pathways to accelerate and reach maximal velocities, followed by the ability to tolerate and clear the associated fatiguing by-products to sustain work (Hirvonen et al., 1992). Nummela, Vuorimaa and Rusko (1992) detailed the physiological responses to the 400m sprint. Blood lactate increased significantly throughout, spiking upwards of 11 mmol/L at the 300m distance (Figure 3).
Figure 3. 400m Lactate Response.
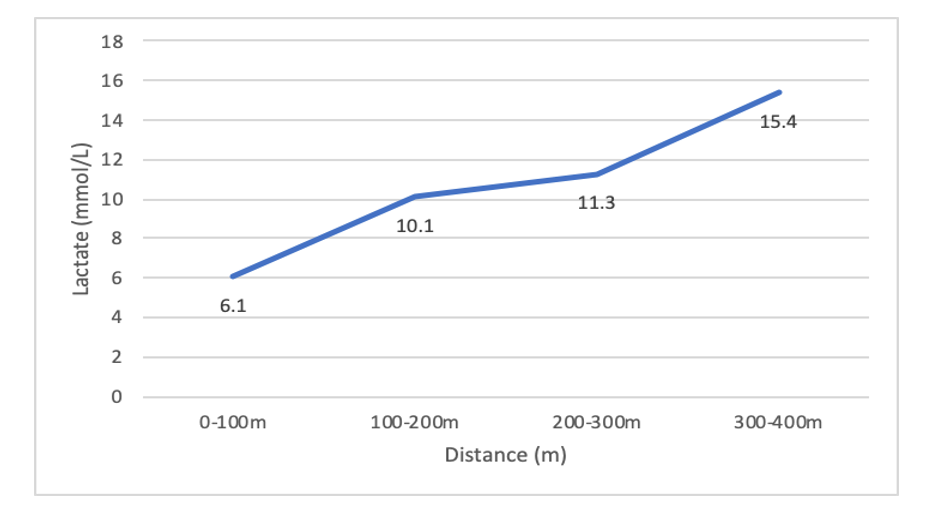
Note: Lactate curve plotted based on data recorded at different points within a 400m race. Adapted from Nummela, Vuorimaa and Rusko (1992).
Contact times linearly increased over the course of the race in coherence with Mann and Murphy (2018) data (Figure 2). Nummela et al. (1992) saw significant increases specifically at two points of the sprint, the 200m and 300m mark. It was hypothesised the increase in contact time at 200m was due to the reduction of phosphocreatine and increased dependence of fast glycolysis for ATP resynthesises. This subsequently leads to reduction in ATP turnover rate and increase of blood lactate as a by-product, which constituted in a further decrease in contact time during the last 100m. Moreover, this was supported by EMG and ground force reaction data. Increased muscle contractility was observed throughout and decreases in ground reaction force were specifically observed in the last 100m. Thus, signifying fatiguability and limitation of fast twitch fibres with the uptake of slow twitch fibres to compensate for contractile failure and reduction in stored elastic energy from the musculotendinous unit. Furthermore, this finding supports the aggressive race pacing strategies demonstrated inresearch from Hanon and Gajer (2009). A race analysis performed by Brice (2018) details the 400m world championship female final in Berlin and assists in conceptualising the physiological responses outlined by Nummela et al. (1992) when measuring individual athletes velocity (Figure 4).
Figure 4. 100m Splits at World Championship Final in Berlin 2009
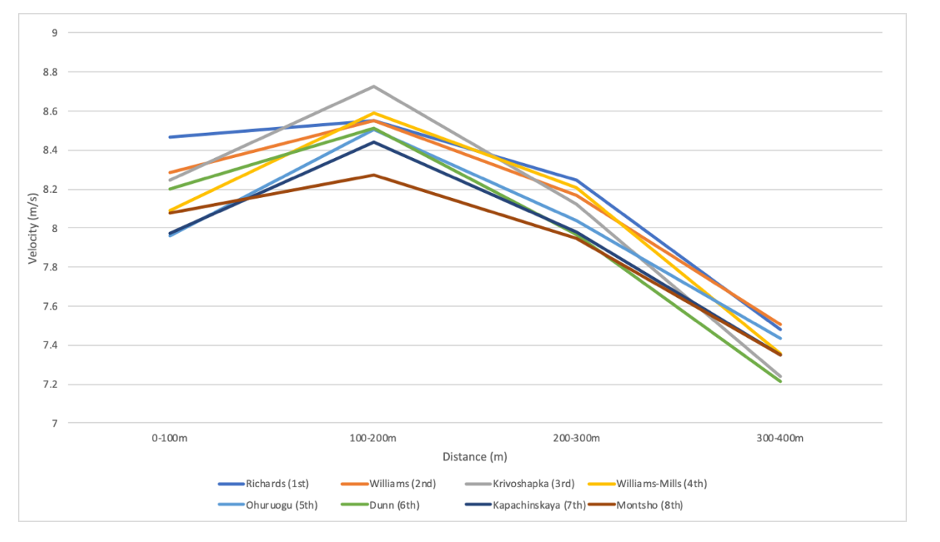
Note: 100m splits calculated and represented as velocity in the 400m world championship final in Berlin 2009. Adapted from Brice (2018).
Ultimately, the resistance of peripheral fatigue is a training adaptation that is categorised by increased oxidative fibre type environment, most notably fast twitch type 2a muscle fibres. Furthermore, increase tolerance to fatiguing metabolites by increasing muscle buffering capability and the ability to shuttle fatiguing metabolites away from the working muscles (Buchheit et al., 2013; Farland et al., 2015; Hanon et al., 2009; Macinnis et al., 2016). To summarise, If the athlete doesn’t have the biological power necessary, they will be unable to maintain optimal mechanics. This can be commonly observed in the last 50m where athletes struggle to maintain efficiency and a breakdown of technique occurs. Everyone slows down, but who slows down the least.
If you made it this far, I applaud you. You've read through a detailed sports analysis (minus the injury data). Perhaps you can spot the problem already. That is, before we even get to the athletes individual needs we see some contrasting physical attributes which are key to maximising performance. The complexity here even for a simple 400m lap around the track is evident. In summary; Success is dependent on acceleration, maximal velocity and the ability to sustain maximal velocity. When exceptional top end speed is vital but so is resistance to fatigue, then race pacing becomes everything. Ultimately, the best strategy is whatever gets the athlete over the line in the quickest time, and that can vary.
If we look back at the data above from Brice (2018), Krivoshapka the Russian athlete who finished 3rd was the fastest and leading at the 200m mark. She was fast, which is a clear KPI, you have to be sufficiently fast but in this case is she too fast for her own good? She had the largest decrement of velocity over the last 200m. Poor race pace or maybe insufficient capacity to resist fatigue leading to the mechanical breakdown perhaps? Was this the difference in a bronze and gold medal? Perhaps if she spent more time in training improving her biological power and increasing her resistance to peripheral fatigue, she may have won. Or perhaps she was nervous and attacked the first 200m too hard? Montsho on the other hand was the slowest over 200m but displays similar consistency in terms of decrement in velocity as race winner Sanya Richards. Didn't go out hard enough or just not fast enough?
In order to know the answer to the questions above you would need to know Krivoshapka and Montsho's strengths and weaknesses (this is where the athlete needs analysis comes in) BUT that only takes us so far. We often only think about strengths and weaknesses in terms of simple biomechanics or physiological markers e.g. force profiles and lactate scores or things that are removed from the sport such as squat strength (at the very general level this probably is enough and maybe fairly obvious markers for improvement) The comparative analysis however is only complete when strategy is considered. 'Is this the best strategy to run faster? How does a differing strategy manipulate the performance?'. You can only answer that with tried and tested robust performance data. You would need to know if the athletes performance here is genuinely consistent and in line with other performances in training and under the stress of competition. That is where the understanding of the sport and the importance of knowing your sport helps. Then and only then do you see if other data corresponds with your findings in order to make the best decision.
The sport tells us what is required, the athlete tells us what they can do, understanding performance strategy tells us what the possibilities are.
References
Blagrove, R. (2015). Strength and conditioning for endurance running. Wiltshire: The Crowood Press. Bosch, F., &Cook, K. (2015). Strength training and coordination: an integrative approach. Rotterdam:
2010 Uitgevers.
Buchheit, M., & Laursen, P. B. (2013). High-Intensity Interval Training, Solutions to the Programming Puzzle. SportsMedicine, 43(10), 927–954.
Cassidy, T. (2004). Understanding sports coaching. Social, cultural & pedagogical foundations of coachingpractice. London: Routledge.
Clark, K. P., & Weyand, P. G. (2014). Are running speeds maximized with simple-spring stance mechanics?Journal of Applied Physiology, 117(6), 604–615.
Cleather, D. (2018). Little black book of training wisdom.
Cormack, S. J., Newton, R. U., & Mcguigan, M. R. (2008). Neuromuscular and Endocrine Responses of Elite Players to an Australian Rules Football Match. International Journal of Sports Physiology and Performance,3(3), 359–374.
Cronin, J., Ogden, T., Lawton, T., & Brughelli, M. (2007). Does Increasing Maximal Strength Improve SprintRunning Performance? Strength and Conditioning Journal, 29(3), 86–95.
Cross, M. R., Brughelli, M., Samozino, P., & Morin, J.-B. (2016). Methods of Power-Force-Velocity ProfilingDuring Sprint Running: A Narrative Review. Sports Medicine, 47(7), 1255–1269.
Dr Paul Brice Consultant Biomechanist Race Analysis: 400m ... (n.d.). Retrieved fromhttps://www.atletiekunie.nl/sites/default/files/userfiles/thema/themadagen/Trainerscongres/Coachcongres_Oktober_2018/Paul Brice - Coaching Conference Race Analysis 400m and 400mh (27.10.18).pdf
Duffield, R., Dawson, B., & Goodman, C. (2005). Energy system contribution to 400-metre and 800- metre track running. Journal of Sports Sciences, 23(3), 299–307.
Edouard, P., Branco, P., & Alonso, J.-M. (2016). Muscle injury is the principal injury type and hamstring muscle injury is the first injury diagnosis during top-level international athletics championships between 2007 and2015. British Journal of Sports Medicine, 50(10), 619–630.
Farland, C. V., Schuette, J., Foster, C., Porcari, J. P., Doberstein, S. T., Harbin, M., … Tuuri, A. (2015). The Effectsof High Intensity Interval Training versus Steady State Training on Aerobic and Anaerobic Capacity. Medicine& Science in Sports & Exercise, 47, 133.
Goodwin, J (2011). Maximum Velocity is When We Can No Longer Accelerate Using biomechanics to informspeed development. Strength and Conditioning, 3-9.
Goodwin, J., Tawiah-Dodoo, J., Waghorn, R., & Wild, J. (2018). Sprint Running. Routledge Handbook of Strength and Conditioning, 473–505.
Grgić, D., Babic, V., & Blažević, I. (2019). Running Dynamics In Male 400M Sprint Event. Series IX Sciences ofHuman Kinetics, 12(61)(2), 41–48.
Hanon, C., & Gajer, B. (2009). Velocity and Stride Parameters of World-Class 400-Meter Athletes Compared With Less Experienced Runners. Journal of Strength and Conditioning Research, 23(2), 524–531.
Heiderscheit, B. C., Hoerth, D. M., Chumanov, E. S., Swanson, S. C., Thelen, B. J., & Thelen, D. G. (2005). Identifying the time of occurrence of a hamstring strain injury during treadmill running: A case study. ClinicalBiomechanics, 20(10), 1072–1078.
Hirvonen, J., Nummela, A., Rusko., Heikki., Rehunen, S., & Härkönen, M. (1992). Fatigue and changes of ATP,creatine phosphate, and lactate during the 400-m sprint. Canadian journal of sport sciences, 17(1), 141-4.
Hill, D. W. (1999). Energy system contributions in middle-distance running events. Journal of Sports Sciences,17(6), 477–483.
Hunter, J. P., Marshall, R. N., & Mcnair, P. J. (2004). Interaction of Step Length and Step Rate during SprintRunning. Medicine & Science in Sports & Exercise, 36(2), 261–271.
Kugler, F., & Janshen, L. (2010). Body position determines propulsive forces in accelerated running. Journal ofBiomechanics, 43(2), 343–348.
Kuitunen, S., Komi, P. V., & Kyröläinen, H. (2002). Knee and ankle joint stiffness in sprint running. Medicine& Science in Sports & Exercise, 34(1), 166–173.
Kyröläinen, H., Avela, J., & Komi, P. V. (2005). Changes in muscle activity with increasing running speed. Journalof Sports Sciences, 23(10), 1101–1109.
Lorenz, D., & Morrison, S. (2015). Current Concepts in Periodization of Strength and Conditioning for the Sports Physical Therapist. The International Journal of Sports Physical Therapy. 10. 734-747.
Macinnis, M. J., & Gibala, M. J. (2016). Physiological adaptations to interval training and the role of exerciseintensity. The Journal of Physiology, 595(9), 2915–2930.
Mann, J. B., Thyfault, J. P., Ivey, P. A., & Sayers, S. P. (2010). The Effect of Autoregulatory Progressive Resistance Exercise vs. Linear Periodization on Strength Improvement in College Athletes. Journal of Strength and Conditioning Research, 24(7), 1718–1723.
Mann, R., Kotmel, J., Herman, J., Johnson, O and Schultz, C. (1984) Kinematic trends in elite sprinters.Sports biomechanics, Academic Publishers.
Mann, R., & Murphy, A. (2018). The mechanics of sprinting and hurdling. Place of publication not identified:publisher not identified.
Mcbride, J. M., Blow, D., Kirby, T. J., Haines, T. L., Dayne, A. M., & Triplett, N. T. (2009). Relationship Between Maximal Squat Strength and Five, Ten, and Forty Yard Sprint Times. Journal of Strength and ConditioningResearch, 23(6), 1633–1636.
Mcguigan, M. R., Doyle, T. L., Newton, M., Edwards, D. J., Nimphius, S., & Newton, R. U. (2006). Eccentric Utilization Ratio: Effect of Sport and Phase of Training. The Journal of Strength and Conditioning Research, 20(4), 992.
Mclean, B. D., Coutts, A. J., Kelly, V., Mcguigan, M. R., & Cormack, S. J. (2010). Neuromuscular, Endocrine, andPerceptual Fatigue Responses During Different Length Between-Match Microcycles in Professional RugbyLeague Players. International Journal of Sports Physiology and Performance, 5(3), 367–383.
Mero, A., Komi, P., & Gregor, R. (1992). Biomechanics of Sprint Running. Sports Medicine, 13(6), 376– 392.
Monte, A., Muollo, V., Nardello, F., & Zamparo, P. (2016). Sprint running: how changes in step frequency affect running mechanics and leg spring behaviour at maximal speed. Journal of Sports Sciences, 35(4), 339–345.
Morin, J.-B., Edouard, P., & Samozino, P. (2011). Technical Ability of Force Application as a Determinant Factor of Sprint Performance. Medicine & Science in Sports & Exercise, 43(9), 1680– 1688.
Morin, J.-B., Slawinski, J., Dorel, S., Villareal, E. S. D., Couturier, A., Samozino, P., … Rabita, G. (2015a).Acceleration capability in elite sprinters and ground impulse: Push more, brake less? Journal of Biomechanics,48(12), 3149–3154.
Morin, J.-B., Gimenez, P., Edouard, P., Arnal, P., Jiménez-Reyes, P., Samozino, P., … Mendiguchia, J. (2015b).Sprint Acceleration Mechanics: The Major Role of Hamstrings in Horizontal Force Production. Frontiers in Physiology, 6.
Nummela, A., Vuorimaa, T., & Rusko, H. (1992). Changes in force production, blood lactate and EMG activity inthe 400‐m sprint. Journal of Sports Sciences, 10(3), 217–228.
Nummela, A., & Rusko, H. (1995). Time Course of Anaerobic and Aerobic Energy Expenditure During Short-TermExhaustive Running in Athletes. International Journal of Sports Medicine, 16(08), 522– 527. Quercetani, R. (2005). A world history of the one lap race: the killer sprint: 400 m., and 4x4 m. relay, - Men and Women (1850-2004). Milan: Sep Editrice.
Rabita, G., Dorel, S., Slawinski, J., Sàez-De-Villarreal, E., Couturier, A., Samozino, P., & Morin, J.-B. (2015).Sprint mechanics in world-class athletes: a new insight into the limits of human locomotion. Scandinavian Journal of Medicine & Science in Sports, 25(5), 583–594.
Slawinski, J., Termoz, N., Rabita, G., Guilhem, G., Dorel, S., Morin, J.-B., & Samozino, P. (2015). How 100-m eventanalyses improve our understanding of world-class mens and womens sprint performance. Scandinavian Journal of Medicine & Science in Sports, 27(1), 45–54.
Slawinski, J., Bonnefoy, A., Levêque, J.-M., Ontanon, G., Riquet, A., Dumas, R., & Chèze, L. (2010). Kinematicand Kinetic Comparisons of Elite and Well-Trained Sprinters During Sprint Start. Journal of Strength and Conditioning Research, 24(4), 896–905.
Sleivert, G., & Taingahue, M. (2004). The relationship between maximal jump-squat power and sprint accelerationin athletes. European Journal of Applied Physiology, 91(1), 46–52. Spencer, M. R., & Gastin, P. B. (2001). Energy system contribution during 200- to 1500-m running in highlytrained athletes. Medicine and Science in Sports and Exercise, 157–162.
Styles, W. J., Matthews, M. J., & Comfort, P. (2016). Effects of Strength Training on Squat and Sprint Performance in Soccer Players. Journal of Strength and Conditioning Research, 30(6), 1534–1539. Thompson, M. A. (2017). Physiological and Biomechanical Mechanisms of Distance Specific Human RunningPerformance. Integrative and Comparative Biology, 57(2), 293–300. Timmons, J. A. (2011). Variability in training-induced skeletal muscle adaptation. Journal of Applied Physiology,110(3), 846–853.
Toyoshima, R., & Sakurai, S. (2016). Kinematic Characteristics of High Step Frequency Sprinters and Long StepLength Sprinters at Top Speed Phase. International Journal of Sport and Health Science, 14(0), 41–50.
Weyand, P. G., Sternlight, D. B., Bellizzi, M. J., & Wright, S. (2000). Faster top running speeds are achieved withgreater ground forces not more rapid leg movements. Journal of Applied Physiology, 89(5), 1991–1999.
Weyand, P. G., Sandell, R. F., Prime, D. N. L., & Bundle, M. W. (2010). The biological limits to running speed areimposed from the ground up. Journal of Applied Physiology, 108(4), 950–961.
Wild, James & Bezodis, Neil & Blagrove, Richard & Bezodis, Ian. (2011). A Biomechanical Comparison ofAccelerative and Maximum Velocity Sprinting: Specific Strength Training Considerations. ProfessionalStrength and Conditioning.
Wisloff, U. (2004). Strong correlation of maximal squat strength with sprint performance and vertical jump heightin elite soccer players. British Journal of Sports Medicine, 38(3), 285–288.
Zouhal, H., Jabbour, G., Jacob, C., Duvigneau, D., Botcazou, M., Abderrahaman, A. B., … Moussa, E. (2010).Anaerobic and Aerobic Energy System Contribution to 400-m Flat and 400-m Hurdles Track Running. Journalof Strength and Conditioning Research, 24(9), 2309–2315.
Čoh, M., & Mackala, K. (2013). Differences Between the Elite and Subelite Sprinters in Kinematic and Dynamic Determinations of Countermovement Jump and Drop Jump. Journal of Strength and Conditioning Research, 27(11), 3021–3027.
Comments